An increasing world population has brought concerns over securing the availability of food, energy, water and bioresources; on another hand the increased greenhouse gases emissions and environmental pollution stressed the importance of addressing food, energy, water and climate as a complex system, considering the interactions between those factors, the so-called Food-Energy-Water nexus [1].
Implementing energy savings, renewable energy and more efficient conversion technologies can have positive socio-economic effects, create employment and if externalities such as health effects are included, even more benefits can be expected [2]. The high demand for fossil fuels replacements, has motivated a significant biogas growth, particularly in the European Union. In the year 2017, the global installed capacity for biogas totalled 16915MW, with more than70% of it being installed in the European Union [3]. In fact, to be able to meet the demand of future consumption and to guarantee sustainable structural changes on the energy supply-chains, promoted the search of alternative energy sources [4]. Innovative approaches within the sustainable development of society will lead to the definition of new systems among the energy supply-chains. One of the possible approaches is to focus on a value chain and establish synergistic interactions between actors, improving productivity and reducing losses and environmental impacts [5]. The food supply chain produces large amounts of waste and therefore is one of the targets of the Commission’s Circular Economy Package [6] to stimulate transition towards a circular economy. To tackle this problem, it is important to face the different stages of the supply chain, including primary production, processing, distribution and consumption [7]. This fact can be an opportunity for R&I addressing methodological developments aiming new valorisation strategies encompassing the Food-Energy-Water challenge [8].
As generally agro-food industries include energy intensive processes the possibility to recover energy from waste generated is a valorisation route that is worth exploring. Being a mature technology, anaerobic digestion (AD) can be used for decentralised energy production, removing barriers for market uptake of this solution. One of the possible bottlenecks regarding AD deployment is the process yield that in some cases is not enough to guarantee its viability [9].
Shifting from AD using one mono-substrate as feedstock to anaerobic co-digestion (AcoD) leads to synergetic effects of multiple substrates increasing processes sustainability [10].
Several works have addressed biogas production from agro-food waste as a sustainable option for waste management and underlined the advantages of AcoD [11]; per example, the anaerobic co-digestion of sewage sludge from wastewater treatment plant (WWTP) and agro-food biowastes (AFW) improved digester stability, methane yield and lead to shorter hydraulic retention time [12]. Many authors have highlighted that co-digestion focused on co-substrates compositions is the key factor, where fat-rich material as co-substrates, can, theoretically, produce more methane from lipids (1,014 L/kg) than from proteins (496 L/kg) or carbohydrates (415 L/kg) [13].
The strategy to promote AcoD at rural/urban level should start by establishing the energy-system boundaries, using criteria that include the availability of substrates/feedstocks for AD and AcoD processes and the options for the use of biogas and digestate, rich in nutrients.
At a rural context, most common substrates are livestock effluents [14] whereas co-substrates include for example agro-food biowastes (AFW). AFW have a wide range of physical-chemical characteristics, but in general show a high level of non-fibre carbohydrates and fat content that could lead to fast acidification during the conversion process. To overcome this drawback, AFW is used as co-substrate in mixtures prepared to maximize synergisms, attenuating the inhibition of harmful compounds and not disrupting digestate quality. Multiple feedstocks used as co-substrates can integrate, at urban level, AD plants using sewage sludge as main substrate to enhance biogas production [15].
Another option to enhance bioconversion process is to pre-treat the substrate and/or co-substrate [16]. Pre-treatments weaken cell wall and structure, allowing for enzymes and methanogens to consume organic compounds inside the cell and facilitating solubilization, through different mechanisms [17]. Pre-treatments may be physical, chemical or biological; or a combination of them [18]. Generally, the pre-treatments classification into various categories, include: Mechanical and Physical (I) [19]; Thermal Hydrolysis or Chemical Alkaline + Thermal Hydrolysis (II); and Filtration (III) to promote the recovery of the liquid phase (AcoD liquor) [20]. The main objective of these pre-treatments is to increase the cellulosic materials hydrolysis, especially AFW containing vegetable and lignocellulosic substrates [21].
This paper presents a systematic analysis of results of AcoD obtained by the authors along a period of more than 5 years. A broad variety of AFW were used as co-substrate either with livestock manure (LM) or with mixed sewage sludge (SS). Whenever found necessary co-substrates were pre-treated to increase soluble organic matter content [22]. Comparing the two studied scenarios, the mono-digestion (reference) and co-digestion trials based on an energy performance indicator (EPI), it was possible to show the percentage of increase in the specific methane yield (SMY) with feeding regime shift applied. This kind of analysis could contribute to set a management strategy for different biowastes flows, improving the sustainability of the energy supply for rural and urban sectors.
The method used in this study included the systematic analysis of 12 scientific articles on bioenergy recovery from AFW at rural/urban level, published by the author’s research group. From each article, data on key operational parameters were compiled accordingly to the main substrate used (LM or SS) to compare AD and AcoD trials. These parameters included organic loading rate (OLR, g VSadded/L·d), potential of hydrogen (pH), relation between soluble chemical oxygen demand and chemical oxygen demand (SCOD/COD), and the ratio between total carbon and total nitrogen (C/N). The SMY indicates the potential bioenergy production for the different AFW studied and to close the circular bioeconomy approach, a simple indicator (EPI) was created to link the conventional and innovative tendencies for the AD and AcoD biotechnology. The characteristics of LM and SS used in the studies are described below.
Livestock manure. The studies report two types of LM: pig slurry (PS) obtained one site system farm, including two types of production systems, closed cycle (CC) and fattening/finishing (FF). The FF slurry collected directly from pit ditches simulate the effluent produced at a fattening/finishing farm unit. The liquid dairy cattle manure (LCM) came from a single dairy farm. The monitoring plan, in the different farm units, was adapted to the improvements implemented in farm units for livestock manure at real scale, taking in account water use efficiency, along eight years period (2012-2019). The pig farms selected, covering a range between 500 and 1,000 breeding sows, reflected the concentrated animal feeding operation (CAFO) in the region [23]. The treatment systems implemented include a storage tank; mechanical solid-liquid separation followed by a lagoon system, where the manure liquid fraction is stored for further utilization, being the most common best available technique (BAT) the agronomic valorisation. All the trials presented were carried out on lab-scale, except for the farm 5 [marked (*) in Table 1]. The mobile pilot unit was installed, at the pig farm unit, in order to demonstrate the biogas production optimization in comparison with the AD reactor implemented in the facility. Table 1 exhibits the identification of livestock farms according to size, number of sows lodged, location and sample collection period.
Farms used to collect the livestock manure samples
Farm Code |
Size § |
Animals |
Location [Region] |
Sampling Period |
1† |
Type 1 |
500 sows |
Ribatejo [38º97’73” N, 8º68’13” W] |
2013-2014 |
2† |
Type 1 |
500 sows |
Ribatejo [38º97’73” N, 8º68’13” W] |
2015-2016 |
3† |
Type 1 |
500 sows |
Ribatejo [38º97’73” N, 8º68’13” W] |
2016-2018 |
4† |
Type 1 |
900 sows |
Ribatejo [39º00’14” N, 8º64’70” W] |
2018-2019 |
5†, * |
Type 1 |
1,000 sows |
Ribatejo [39º28’44” N, 8º87’69” W] |
2012-2014 |
6‡ |
Type 2 |
120 cows |
Lisbon [38º57’24” N, 9º08’10” W] |
2013-2014 |
† One site system pig farm; * Biogas plant installed on farm; ‡ Dairy cow farm; §According to industrial emissions Directive 2010/75/EC (24 November 2010) and Portuguese Livestock Activity Directive 2013/81/PT. |
Mixed sewage sludge. The sampling of SS was performed in three Portuguese urban WWTP located in the Lisbon Region (Portugal) covering a range of treatment capacities (13,000 to 60,000 m3/d). The treatment process includes activated sludge system coupled with anaerobic sludge digestion. The water line comprises primary, secondary and tertiary treatments, with biological process for nitrogen removal, according with the legal requirements limits to allow the effluent to be discharge in the water body (Tagus River); the sludge line integrates the following unit processes: pre-thickening, anaerobic digestion, post-thickening and mechanical dewatering by centrifugation.
Table 2 exhibits the classification of plants according to size, inhabitant’s equivalent, location and sample collection period.
Wastewater treatment plants selected for sampling mixed sewage sludge
WWTP Code |
Size § |
IE † |
Location [Region] |
Sampling Period |
1 |
M |
67,000 |
Lisbon [38º37’55” N, 9º04’19” W] |
2014-2016 |
2 |
M/H |
210,000 |
Lisbon [38º43’55” N, 9º07’50” W] |
2015-2016 |
3 |
M/H |
210,000 |
Lisbon [38º43’55” N, 9º07’50” W] |
2016-2017 |
4 |
M/H |
210,000 |
Lisbon [38º43’55” N, 9º07’50” W] |
2016-2017 |
5 |
M/H |
210,000 |
Lisbon [38º43’55” N, 9º07’50” W] |
2017-2018 |
6 |
M/H |
250,000 |
Lisbon [38º49’13” N, 9º08’59” W] |
2017-2018 |
§M – medium; M/H – medium/high; † IE – inhabitant’s equivalent. |
The different types of AFW, for the AcoD experimental trials, were selected, taking in account the synergetic effect with the substrate, mainly their chemical composition to balance the feed mixtures and the Bio-CH4 enhancement. To simplify the discussion of the data obtained, from the studies reviewed and compared according to the AFW bioenergy potential, the five broad categories selected are: (a) fruit and vegetable biowaste (FVW), (b) fish canning industry (FCI), (c) horse manure (HM), (d) coffee biowastes (CW), and (e) non-edible crops (NEC). The first category, fruit and vegetable biowaste (FVW) includes: non-marketable pears, pear biowaste (PW), orange peel biowaste (OP) and carrots peel biowaste (CP), and pineapple peel biowaste (PPW); category b) includes waste sardine oil (WSO); category c), horse manure (HM); category d), coffee biowastes (CW) includes: coffee grounds (CG) and exhausted coffee biowaste (ECB) resulting from the water-pressured extraction process to produce soluble coffee and coffee drinks; and category e), non-edible crops (NEC), that includes elephant grass “Pennisetum purpureum” (EG) and duckweed biomass (DB).
Considering the characteristics of the five AFW categories, different pre-treatment’s combinations were employed to enhance energy availability, improving biodegradability and maximizing liquor recovery. Biodegradability of co-substrates improved by reducing the macromolecule dimensions, breaking bacteria cell walls with release of intracellular organic matter led to a higher SCOD/COD and C/N ratio, contributing for the pH adjustment for the most suitable values recommended for AD process.
Figure 1 presents a chart diagram (up-to-bottom), where the different steps applied to AFW illustrate the routes followed to obtain the final feed material to be mixed with the substrates. After defining the five categories, already described, the following step was the pre-treatment selection, attending to the synergetic effects with the substrates (LM and SS) from the different categories. The pre-treatments applied, alone or in combination were: I-“Mechanical and Physical”; II-“Thermal Hydrolysis” or “Chemical Alkaline + Thermal Hydrolysis”; III-“Filtration”. The final step shows the liquor obtained for AcoD trials designed, respectively by: PLF (pear liquid fraction); SOL (sieved orange peel liquor); LCP (liquid carrot peel); PPL: (pineapple peel liquor); WSO (waste sardine oil); SHM: sieved horse manure; LCG: liquor coffee ground; LECB: liquid exhausted coffee biowaste; EGL: elephant grass liquor; DB: duckweed biomass.
Chart diagram (up-to-bottom) of the different steps applied to AFW
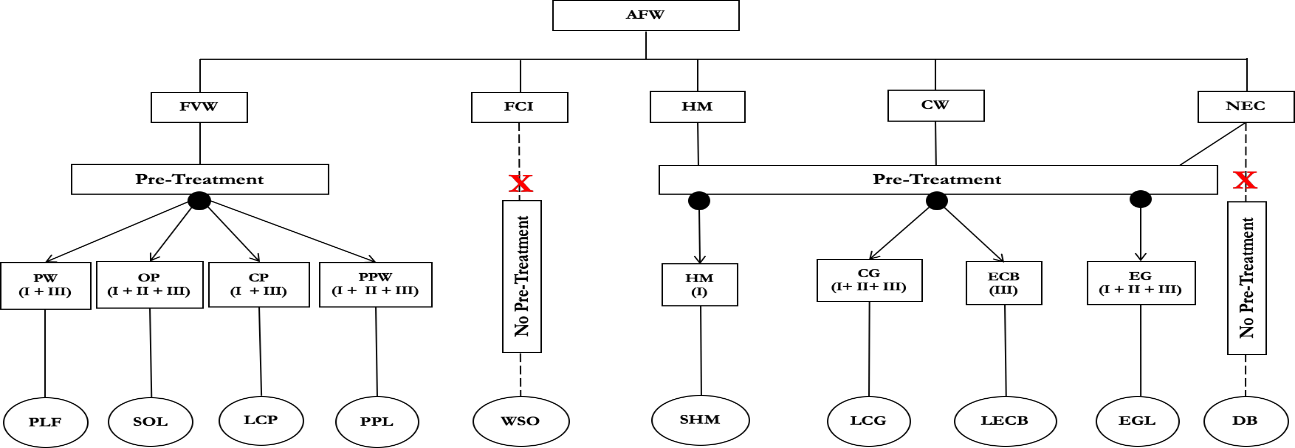
Figure 2 and Figure 3 show, respectively, the layout and images of the experimental AcoD units used in the developed trials, including two lab-scale units [Continuous Stirred Tank Reactor (CSTR)]: a 6.0 L (Unit I) and 12.0 L (Unit II) and a mobile pilot-scale unit (CSTR): 2.0 m3 (Unit III). All the trials were performed under mesophilic conditions (37±1ºC).
Layouts: a) experimental AcoD-lab Unit I; b) experimental AcoD-lab Unit II; c) AcoD-mobile pilot Unit III
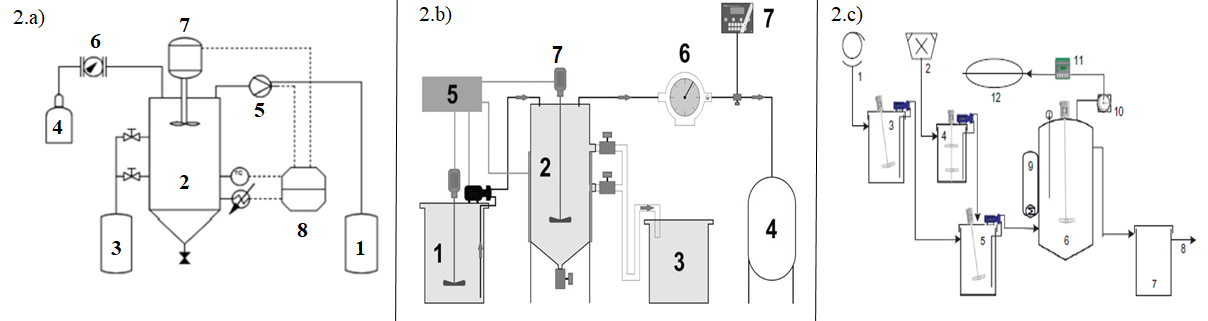
Legend: a) 1: feeding mixture tank; 2: continuous stirred tank reactor (CSTR); 3: digestate tank; 4: gas holder; 5: feeding pump; 6: gas meter; 7: gas analyser; 8: PLC system. b) 1: feeding mixture tank; 2: continuous stirred tank reactor (CSTR); 3: digestate tank; 4: gas holder; 5: feeding pump; 6: gas meter; 7: gas analyser; c) 1: pig slurry screener, 2: physical conditioner of co-substrates, 3: pig slurry tank, 4: co-substrates tank, 5: mixing/influent tank, 6: digester with heating system, 7: digestate tank, 8: digested effluent, 9: hot water, 10: gas meter, 11: gas analyser, 12: gas holder.
a) Experimental AcoD lab Unit I; b) Experimental AcoD lab Unit II; c) Mobile pilot AcoD Unit III
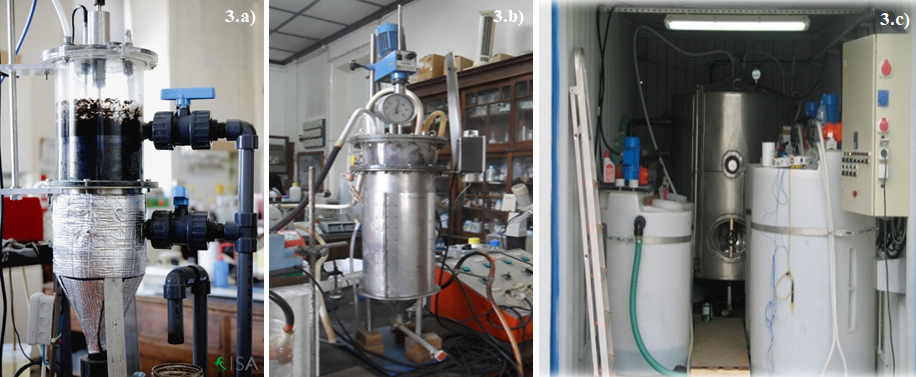
The analytical procedures adopted in the 12 revised articles were common. The following parameters were determined in LM, SS and AFW, feeding-mixtures and digestate streams, in accordance with the standard methods [24]: pH, electrical conductivity (EC), total and volatile solids (TS, VS), volatile suspended solids (VSS), COD and SCOD, total alkalinity (TA), total Kjeldahl nitrogen (TKN) and total ammonium nitrogen (TAN). Total organic carbon (TOC) was also determined [25]. The C/N ratio value obtained respectively, dividing the TOC by the TKN. All analytical determinations performed in triplicate, with analytical grade reagents (≥ 99% purity), were carried out. The AcoD performance was monitored following several operational parameters, methane content (percentage) and specific methane yield (SMY, mLCH4/gVSadded). The reactor stability has been controlled by monitoring the pH and TA in the digestate. Additionally, the ratio between feedstock organic load (expressed in COD) and the amount of biomass inside the bioreactor (expressed in SSV), designed by specific energy loading rate [SELR (d-1)] was calculated [26]. The integration of these two procedures allows the evaluation of the reactor stability conditions.
The main results achieved in the studies performed for both scenarios (mono and co-digestion) are presented below, highlighting that the EPI is a key factor to set a management strategy for different biowaste flows, improving the sustainability of the energy supply for rural and urban sectors.
The results obtained for AD trials under mono-digestion using LM or SS (reference scenario) are compiled in Table 3 and Table 4, respectively.
Mono-digestion AD trials performance, using livestock manure as substrate
Substrate |
Operational Conditions |
Reference |
|||||
IN |
OUT |
||||||
HRT |
OLR |
pH‡ |
SCOD/COD |
C/N |
SMY |
||
LM |
[days] |
[gVS/Lreactor·d]‡ |
[%] |
[mLCH4/gVSadded]‡ |
|||
Farm 1§ |
10 |
0.46±0.10 |
7.8±0.1 |
n.d |
2 |
93±4 |
[27] |
Farm 2§ |
16 |
0.78±0.10 |
7.3±0.1 |
42 |
5 |
110±6 |
[28] |
Farm 3§ |
23 |
0.56±0.11 |
7.1±0.2 |
47 |
3 |
87±15 |
[29] |
Farm 4§ |
16 |
1.50±0.02 |
7.6±0.1 |
27 |
5 |
310±15 |
[30] |
Farm 5§, * |
16 |
1.01±0.08 |
7.6±0.2 |
n.d |
3 |
435±20 |
[31] |
Farm 6† |
14 |
1.14±0.12 |
7.0±0.3 |
n.d |
12 |
113±8 |
[32] |
‡ mean±SD;.n.d: not determined; §: PS as substrate; †: LCM as substrate; * Biogas plant installed on farm. |
Analysing Table 3, and according to the codes presented on Table 1 for LM substrates, the PS from farms 1 to 5 show a pH average value of 7.50.3, which is in the range expected for this type of substrate [33] and referred as ideal in literature for AD process [34]. The LCM exhibited a pH value of 7.00.3, which is also in accordance with data published [35]. The SCOD/COD ratio presented an average value of 38.710.4%, that is in accordance with other studies reported in the literature [36]. Pig slurry from farm 4 (FF) had higher TS content and lower biodegradability as consequence of the increasing presence of non-soluble compounds (food waste, dietary fibre which cannot be digested by the host, pig bristles). The results for reference scenario showed a low to medium C/N ratio, 2 to 5 for PS and 12 for LCM what is lower than that recommended for the AD process [37]. The average value of the SMY obtained for PS substrate (farm 1 to 3) was 925 mL CH4/gVSadded, which is in accordance with data presented by other authors [38]. The difference observed in farm 4 (three times more) could be related to the higher TS of the slurry used as obtained in other authors studies [39]. The highest value (435±20 mL CH4/gVSadded) obtained for the PS sample collected at farm 5 could be explained once the experimental trial was implemented in situ with AcoD-mobile pilot Unit III. The SMY value obtained for LCM (113±8 mL CH4/gVSadded), was in the range of the ones referred by other researchers [35].
Mono-digestion AD trials performance, using mixed sewage sludge as substrate
Substrate |
Operational Conditions |
Reference |
|||||
IN |
OUT |
||||||
HRT |
OLR |
pH ‡ |
SCOD/COD |
C/N |
SMY |
||
SS |
[days] |
[gVS/L reactor· d] ‡ |
[%] |
[mLCH 4 /gVS added ] ‡ |
|||
WWTP 1§ |
15 |
1.65±0.15 |
5.8±0.2 |
10 |
9 |
246±10 |
[40] [40] |
WWTP 1† |
15 |
1.50±0.10 |
5.5±0.1 |
7 |
14 |
422±20 |
|
WWTP 2§ |
15 |
1.13±0.05 |
6.6±0.4 |
7 |
6 |
130±7 |
[41] |
WWTP 3§ |
15 |
1.45±0.10 |
6.1±0.4 |
4 |
9 |
100±5 |
[42] |
WWTP 4§ |
15 |
1.00±0.05 |
5.6±0.4 |
2 |
8 |
79±4 |
[43] |
WWTP 5§ |
17 |
0.89±0.23 |
6.0±0.4 |
2 |
7 |
122±52 |
[44] |
WWTP 6§ |
15 |
1.41±0.05 |
6.2±0.6 |
n.d |
6 |
100±2 |
[45] |
‡mean±SD; n.d: not determined; §: WWTP with 40% of primary sludge and 60% of conventional activated sludge; †: WWTP with 60% of primary sludge and 40% of conventional activated sludge. |
Regarding the results for SS substrate presented in Table 4 for the WWTPs 1 to 6, the pH values ranged from 5.5±0.1 to 6.6±0.4, which is lower than the recommended to AD process [46]; the SCOD/COD ratio ranged from 2% to 10% indicating the presence of soluble intermediate compounds with low biodegradability, this fact can be a drawback for the use of SS as an AD substrate, as it shows a high content of particulate organic matter [47]. The values obtained for C/N ratio, between 6 and 14, are in accordance with the values referred in the literature [46]. The SMY average value for SS (40:60) was 13060 mL CH4/gVSadded, which is in accordance with studies performed with this substrate [48]. The highest SMY value (422±20 mL CH4/gVSadded), obtained for the SS from WWTP 1 (60:40) in comparison with WWTP 2 can be explained, by the increase of primary sludge percentage in the feedstock mixture.
The data obtained under co-digestion conditions was grouped according to the substrates and co-substrates used and presented in Table 5 (LM) and Table 6 (SS), respectively.
Results obtained regarding AcoD trials with livestock manure used as substrate
Operational Conditions |
|||||||||
Subs. |
Co-subs. |
Feedstock [v:v] |
IN |
OUT |
Ref. |
||||
OLR [gVS/Lreactor.d]‡ |
pH‡ |
SCOD/COD [%] |
C/N |
SMY [mLCH4/gVSadded]‡ |
EPI [%] |
||||
PS |
DB |
90:10† |
0.67±0.10 |
7.8±0.1 |
n.d. |
3 |
131±8 |
41 |
[27] |
PS |
LECB |
70:30 |
2.00±0.20 |
6.2±0.2 |
55 |
13 |
341±10 |
210 |
[28] |
PS |
SHM |
80:20 |
0.61±0.25 |
7.8±0.1 |
25 |
5 |
216±12 |
150 |
[29] |
PS |
PPL |
80:20 |
1.45±0.02 |
7.2±0.2 |
52 |
10 |
560±15 |
81 |
[30] |
PS |
WSO |
95:5 |
3.20±0.10 |
n.d |
n.d |
13 |
570±20 |
31 |
[31] |
LCM |
PLF |
25:75 |
1.30±0.03 |
5.5±0.1 |
n.d |
25 |
390±2 |
250 |
[32] |
‡ mean±SD; n.d: not determined; † feedstock (v:m). |
As it can be seen the addition of AFW to different substrates (LM, SS) improved the hydrolysis phase, which is considered the rate and stage-limiting step of AD process [49]. In general terms, LM and SS have a rich content in proteins [48], linked to the lowest hydrolysis rate and biogas yield. The addition of the liquors obtained from the co-substrates, in the form of simple biodegradable matter, accelerated the microbial growth and the hydrolysis rate, contributing for the acidification and methanogenesis steps, resulting in a higher potential biogas production. This enhancement can be predicted by the feeding mixture SCOD/COD ratio applied to AcoD trials [17].
The EPI value allows a simple comparison of the SMY values obtained in AD and AcoD experimental assays. As seen in Table 5 (for LM) the EPI indicator ranged from 30% to 250% showing the advantages of ensuring the stability of AD process, by promoting a suitable C/N ratio and buffer capacity compared with mono-digestion trials (Table 3) [39].
Results obtained regarding AcoD trials with mixed sewage sludge used as substrate
Operational Conditions |
|||||||||
Subs. |
Co-subs. |
Feedstock [v:v] |
IN |
OUT |
Ref. |
||||
OLR [gVS/Lreactor.d]‡ |
pH‡ |
SCOD/COD [%] |
C/N |
SMY [mLCH4/gVSadded]‡ |
EPI [%] |
||||
SS |
EGL |
50:50 |
1.10±0.07 |
7.3±0.5 |
53 |
11 |
210±10 |
62 |
[41] |
SS |
SOL |
70:30 |
1.80±0.31 |
5.2±0.3 |
39 |
15 |
301±15 |
200 |
[42] |
SS |
LCP+LCG |
45:35:20 |
1.38±0.3 |
7.7±0.6 |
34 |
15 |
505±30 |
539 |
[43] |
SS |
LECB |
80:20 |
0.99±0.25 |
4.9±0.2 |
24 |
11 |
276±49 |
126 |
[44] |
SS |
LCP+LCG |
40:35:25 |
1.12±0.3 |
7.6±0.2 |
n.d |
18 |
390±25 |
290 |
[45] |
‡ mean±SD; n.d: not determined. |
For trials with SS (Table 6) the EPI indicator’s values ranged from 62% to 539%, showing an increase of energy recovery, which improves the bioenergy supply and reduces the overall operational costs [50].Nowadays, the main goals established by the WWTP (medium; medium/high size) located in urban areas are to improve environmental sustainability and energy self-sufficiency. The results obtained in our studies using AFW from different sources and with a broad physical-chemical composition confirmed that they should be integrated with SS substrate towards a transition to a circular waste-based-bioeconomy [8].
The AD technology is not successfully implemented in livestock sector in Portugal. However, the producers are starting to change their behaviour, mainly due to the EU targets for the renewable energy systems linked with sustainable development scenarios.
Wastewater is usually considered as a potential energy source. The main energy contributor in a WWTP is the biogas produced in the digester. The use of biogas for digester heating and electricity generation is viewed as a sustainable way of recovering energy at WWTP, with subsequent sludge reduction. A WWTP with pre-settling and sludge digestion on average consumes 40% less net energy compared to that without sludge digestion. Meanwhile, many WWTP do not use the total active volume of the digester. In this sense, the addition of AFW, easily biodegradable, for anaerobic co-processing with SS can contribute to overcome the drawbacks associated with SS poor energy recovery efficiency.
Synergetic interactions between substrates supply (LM and SS) and the co-substrates (AFW) should be considered to the food-energy-water nexus [5], to improve productivity and minimize losses in the production chain. Spatial scales should receive more attention [51]. From a market and industry perspective, successful food-energy-water nexus projects need a transdisciplinary approach, ecological technology practices, and to promote sustainable supply chains. Due to many interrelationships of the nexus, R&I opportunities may include methodological developments, social concerns, performance indicator-based systems, and meta-social evolutions in technology and policy [52].
This work intended to contribute to an integrated management of animal farming wastes (LM) and AFW for biogas production providing a profitable solution. On the other hand, the AcoD of SS with AFW improved the quality of biogas due to the selection of a hydrolytic pre-treatment, which facilitates the microorganism access to plant fibre structures reducing hydraulic retention time (HRT) in anaerobic digesters. This also demonstrates the synergies that lead to the improvement in biogas production, reinforcing WWTP’s potential to become a surplus producer of renewable energy in the future.
This study provided a simple basis to design feasible solutions for bioenergy production from livestock manure, mixed sewage sludge and agro-food biowastes. Considering the energy performance indicator (EPI) proposed in this paper, co-digestion significantly improves process performance leading to considerably higher bioenergy recovery.
A stable process operation was observed for co-digestion trials with livestock manure, at an organic loading rate of up to 1.30±0.03 gVS/Lreactor·d, with the highest EPI of 250% considering a hydraulic retention time of 14 days. Regarding co-digestion trials with mixed sewage sludge, at an organic loading rate of up to 1.38±0.3 gVS/Lreactor·d, the highest EPI was 539% for a hydraulic retention time of 15 days. It is important to highlight the positive synergetic effect of the addition of two co-substrates (LCP+LCG) to improve biodegradation feedstock, resulting in a higher methane yield when compared with sewage sludge as mono-substrate.
Agro-food biowastes are a promising co-digestion substrate what contributes to the valorisation of those biowastes for bioenergy recovery (Bio-CH4) in the framework of bioeconomy perspectives.
The Linking Landscape, Environment, Agriculture and Food (LEAF) research unit (UID/AGR/04129/2013) supported this work.
AcoD |
Anaerobic co-Digestion |
AD |
Anaerobic Digestion |
AFW |
Agro-food Biowaste |
BAT |
Best Available Technique |
CAFO |
Concentrated Animal Feeding Operation |
CC |
Closed Cycle |
CG |
Coffee Grounds |
COD |
Chemical Oxygen Demand |
CP |
Carrots Peel |
CSTR |
Continuous Stirred Tank Reactor |
CW |
Coffee Biowastes |
DB |
Duckweed Biomass |
EC |
Electrical Conductivity |
ECB |
Exhausted Coffee Biowaste |
EG |
Elephant Grass |
EGL |
Elephant Grass Liquor |
EPI |
Energy Performance Indicator |
FCI |
Fish Canning Industry |
FF |
Fattening/Finishing |
FVW |
Fruit and Vegetable Biowaste |
HM |
Horse Manure |
HRT |
Hydraulic Retention Time |
IE |
Inhabitants Equivalent |
LCG |
Liquor Coffee Grounds |
LCM |
Dairy Cattle Manure |
LCP |
Liquor Carrot Peel |
LEAF |
Linking landscape, Environment, Agriculture and Food |
LECB |
Liquid Exhausted Coffee Biowaste |
LM |
Livestock Manure |
NEC |
Non-Edible Crops |
OLR |
Organic Loading Rate |
OP |
Orange Peel Biowaste |
PLF |
Pear Liquid Fraction |
PPL |
Pineapple Peel Liquor |
PPW |
Pineapple Peel Biowaste |
PS |
Pig Slurry |
PW |
Pear Biowaste |
SCOD |
Soluble Chemical Oxygen Demand |
SELR |
Specific Energy Loading Rate |
SHM |
Sieved Horse Manure |
SMY |
Specific Methane Yield |
SOL |
Sieved Orange Peel Liquor |
SS |
Mixed Sewage Sludge |
TA |
Total Alkalinity |
TAN |
Total Ammonium Nitrogen |
TKN |
Total Kjeldahl Nitrogen |
TOC |
Total Organic Carbon |
TS |
Total Solids |
VS |
Volatile Solids |
VSS |
Volatile Suspended Solids |
WSO |
Wasted Sardine Oil |
WWTP |
Wastewater treatment plant |
From state to system: Financialization and the water-energy-food-climate nexus ,Geoforum , Vol. 91 (June 2017),pp 151-159 , 2018, https://doi.org/10.1016/j.geoforum.2018.03.001
, Biogas: Developments and perspectives in Europe ,Renewable Energy , Vol. 129 ,pp 457-472 , 2018, https://doi.org/10.1016/j.renene.2018.03.006
, - Renewable Capacity Statistics 2018 , 2018
A review of anaerobic digestion systems for biodegradable waste: Configurations, operating parameters, and current trends ,Environmental Engineering Research , Vol. 25 (1),pp 1-17 , 2020, https://doi.org/10.4491/eer.2018.334
, Biorefineries and the food, energy, water nexus — towards a whole systems approach to design and planning ,Current Opinion in Chemical Engineering , Vol. 18 (September),pp 16-22 , 2017, https://doi.org/10.1016/j.coche.2017.08.003
, Anaerobic digestion for bioenergy production: Global status, environmental and techno-economic implications, and government policies ,Bioresource Technology , Vol. 247 (July 2017),pp 1015-1026 , 2018, https://doi.org/10.1016/j.biortech.2017.09.004
, Introducing a Circular Economy: New Thinking with New Managerial and Policy Implications ,California Management Review , Vol. 60 (3),pp 5-19 , 2018, https://doi.org/10.1177/0008125618764691
, Transdisciplinarity and the food energy and water nexus: Ecological modernization and supply chain sustainability perspectives ,Resources, Conservation and Recycling , Vol. 133 (December 2017),pp 309-319 , 2018, https://doi.org/10.1016/j.resconrec.2018.01.001
, A comprehensive review on food waste anaerobic digestion: Research updates and tendencies ,Bioresource Technology , Vol. 247 (July 2017),pp 1069-1076 , 2018, https://doi.org/10.1016/j.biortech.2017.09.109
, Achievements and perspectives of anaerobic co-digestion: A review ,Journal of Cleaner Production , Vol. 194 (1),pp 359-371 , 2018, https://doi.org/10.1016/j.jclepro.2018.05.155
, Anaerobic co-digestion of sludge and organic food waste-performance, inhibition, and impact on the microbial community ,Energies , Vol. 11 (9), 2018, https://doi.org/10.3390/en11092325
, Anaerobic co-digestion of municipal sewage sludge and fruit/vegetable waste: effect of different mixtures on digester stability and methane yield ,Journal of Environmental Science and Health - Part A Toxic/Hazardous Substances and Environmental Engineering , Vol. 54 (7),pp 628-634 , 2019, https://doi.org/10.1080/10934529.2019.1579523
, - , , Biofuels: Alternative Feedstocks and Conversion Processes for the Production of Liquid and Gaseous Biofuels, 2019
Anaerobic co-digestion of animal manures and lignocellulosic residues as a potent approach for sustainable biogas production ,Renewable and Sustainable Energy Reviews , Vol. 79 (May),pp 308-322 , 2017, https://doi.org/10.1016/j.rser.2017.05.137
, Methane potential of fruit and vegetable waste: an evaluation of the semi-continuous anaerobic mono-digestion ,Environmental Technology (United Kingdom) , Vol. 41 (7),pp 921-930 , 2020, https://doi.org/10.1080/09593330.2018.1515262
, Overview of pretreatment strategies for enhancing sewage sludge disintegration and subsequent anaerobic digestion: Current advances, full-scale application and future perspectives ,Renewable and Sustainable Energy Reviews , Vol. 69 (March 2016),pp 559-577 , 2017, https://doi.org/10.1016/j.rser.2016.11.187
, Reviewing the anaerobic digestion of food waste: From waste generation and anaerobic process to its perspectives ,Applied Sciences (Switzerland) , Vol. 8 (10), 2018, https://doi.org/10.3390/app8101804
, Effect of chemical pretreatment using sulfuric acid on biogas production from water hyacinth and kinetics ,Renewable Energy , Vol. 132 ,pp 335-350 , 2019, https://doi.org/10.1016/j.renene.2018.07.121
, Reviewing the anaerobic digestion and co-digestion process of food waste from the perspectives on biogas production performance and environmental impacts ,Environmental Science and Pollution Research , Vol. 23 (24),pp 24435-24450 , 2016, https://doi.org/10.1007/s11356-016-7159-2
, Pretreatment and co-digestion of wastewater sludge for biogas production: Recent research advances and trends ,Renewable and Sustainable Energy Reviews , Vol. 114 (July),pp 109287 , 2019, https://doi.org/10.1016/j.rser.2019.109287
, - , Effect of sulfuric acid pretreatment on biogas production from Salvinia molesta, Journal of Environmental Chemical Engineering, 2019
Innovative pretreatment strategies for biogas production ,Bioresource Technology , Vol. 224 ,pp 13-24 , 2017, https://doi.org/10.1016/j.biortech.2016.11.083
, - , , Portugal Statistics Yearbook 2017 (in Portuguese), 2017
- , , Standard Methods for the Examination of Water and Wastewater, 2017
Anaerobic co-digestion of swine manure with energy crop residues ,Biotechnology and Bioprocess Engineering , Vol. 16 (5),pp 1044-1052 , 2011, https://doi.org/10.1007/s12257-011-0117-4
, - , Anaerobic Digestion and Energy Recovery from Food Waste, Proceedings of the Water Environment Federation, 2012
Potential of duckweed for swine wastewater nutrient removal and biomass valorisation through anaerobic co-digestion ,Journal of Sustainable Development of Energy, Water and Environment Systems , Vol. 5 (2),pp 127-138 , 2017, https://doi.org/10.13044/j.sdewes.d5.0137
, - , Soluble Coffee Grounds Valorisation by Anaerobic Co-Digestion with Pig Slurry (in Portuguese), 2016
Enhanced biogas production from anaerobic co-digestion of pig slurry and horse manure with mechanical pre-treatment ,Environmental Technology (United Kingdom) , Vol. 40 (10),pp 1289-1297 , 2019, https://doi.org/10.1080/09593330.2017.1420698
, Performance of Anaerobic Co-digestion of Pig Slurry with Pineapple (Ananas comosus) Bio-waste Residues ,Waste and Biomass Valorization , (0123456789), 2020, https://doi.org/10.1007/s12649-020-00959-w
, Utilization of wasted sardine oil as co-substrate with pig slurry for biogas production - A pilot experience of decentralized industrial organic waste management in a Portuguese pig farm ,Bioresource Technology , Vol. 116 ,pp 285-289 , 2012, https://doi.org/10.1016/j.biortech.2012.03.063
, Anaerobic co-digestion of dairy cattle manure and pear waste ,Bioresource Technology , Vol. 164 ,pp 420-423 , 2014, https://doi.org/10.1016/j.biortech.2014.04.110
, Simultaneous biogas and biogas slurry production from co-digestion of pig manure and corn straw: Performance optimization and microbial community shift ,Bioresource Technology , Vol. 282 (January),pp 37-47 , 2019, https://doi.org/10.1016/j.biortech.2019.02.122
, Effects of high-pressure anaerobic digestion up to 30 bar on pH-value, production kinetics and specific methane yield ,Energy , Vol. 138 ,pp 659-667 , 2017, https://doi.org/10.1016/j.energy.2017.07.095
, Anaerobic co-digestion process for biogas production: Progress, challenges and perspectives ,Renewable and Sustainable Energy Reviews , Vol. 76 (March 2016),pp 1485-1496 , 2017, https://doi.org/10.1016/j.rser.2016.11.184
, Methane production and characteristics of the microbial community in the co-digestion of spent mushroom substrate with dairy manure ,Bioresource Technology , Vol. 250 (November 2017),pp 611-620 , 2018, https://doi.org/10.1016/j.biortech.2017.11.088
, Biochemical methane potentials and organic matter degradation of swine slurry under mesophilic anaerobic digestion ,Indian Journal of Science and Technology , Vol. 9 (6), 2016, https://doi.org/10.17485/ijst/2016/v9i6/77183
, Energy production from piggery waste using anaerobic digestion: Current status and potential in Cyprus ,Renewable Energy , Vol. 71 ,pp 263-270 , 2014, https://doi.org/10.1016/j.renene.2014.05.003
, Anaerobic Co-digestion of Pig Manure with Dried Maize Straw ,BioResources , Vol. 11 (4),pp 8914-8928 , 2016, https://doi.org/10.15376/biores.11.4.8914-8928
, Study of different ratios of primary and waste activated sludges to enhance the methane yield ,Water and Environment Journal , Vol. 30 (3-4),pp 203-210 , 2016, https://doi.org/10.1111/wej.12188
, Water-energy nexus: Anaerobic co-digestion with elephant grass hydrolyzate ,Journal of Environmental Management , Vol. 181 ,pp 48-53 , 2016, https://doi.org/10.1016/j.jenvman.2016.06.012
, Effect of Minimizing d-Limonene Compound on Anaerobic Co-digestion Feeding Mixtures to Improve Methane Yield ,Waste and Biomass Valorization , Vol. 10 (1),pp 75-83 , 2019, https://doi.org/10.1007/s12649-017-0048-1
, - , The challenge in bioenergy sector through the anaerobic co-digestion of different biowastes, 5th International Conference on Water Energy and Environment (ICWEE), 2017
Enhancement of Sewage Sludge Bioconversion to Methane by the Addition of Exhausted Coffee Biowaste Liquid Fraction ,Waste and Biomass Valorization , Vol. 11 (3),pp 1125-1130 , 2020, https://doi.org/10.1007/s12649-018-0486-4
, - , Increasing self-sufficiency of a wastewater treatment plant with integrated implementation of anaerobic co-digestion and photovoltaics, 2018 International Young Engineers Forum (YEF-ECE), 2018
Recent trends in anaerobic co-digestion: Fat, oil, and grease (FOG) for enhanced biomethanation ,Progress in Energy and Combustion Science , Vol. 70 ,pp 22-42 , 2019, https://doi.org/10.1016/j.pecs.2018.08.002
, Fate of proteins of waste activated sludge during thermal alkali pretreatment in terms of sludge protein recovery ,Frontiers of Environmental Science and Engineering , Vol. 13 (2), 2019, https://doi.org/10.1007/s11783-019-1114-7
, Enhancement of biogas production from sewage sludge in a wastewater treatment plant: Evaluation of pretreatment techniques and co-digestion under mesophilic and thermophilic conditions ,Energy , Vol. 157 ,pp 707-717 , 2018, https://doi.org/10.1016/j.energy.2018.06.003
, Estimating anaerobic biodegradability indicators for waste activated sludge ,Chemical Engineering Journal , Vol. 160 (2),pp 488-496 , 2010, https://doi.org/10.1016/j.cej.2010.03.059
, Environmental sustainability of an energy self-sufficient sewage treatment plant: Improvements through DEMON and co-digestion ,Water Research , Vol. 74 ,pp 166-179 , 2015, https://doi.org/10.1016/j.watres.2015.02.013
, Conceptual vision of bioenergy sector development in Mediterranean regions based on decentralized thermochemical systems ,Sustainable Energy Technologies and Assessments , Vol. 23 (April 2015),pp 33-47 , 2017, https://doi.org/10.1016/j.seta.2017.09.006
, Unpacking the nexus: Different spatial scales for water, food and energy ,Global Environmental Change , Vol. 48 (November 2017),pp 22-31 , 2018, https://doi.org/10.1016/j.gloenvcha.2017.11.005
,